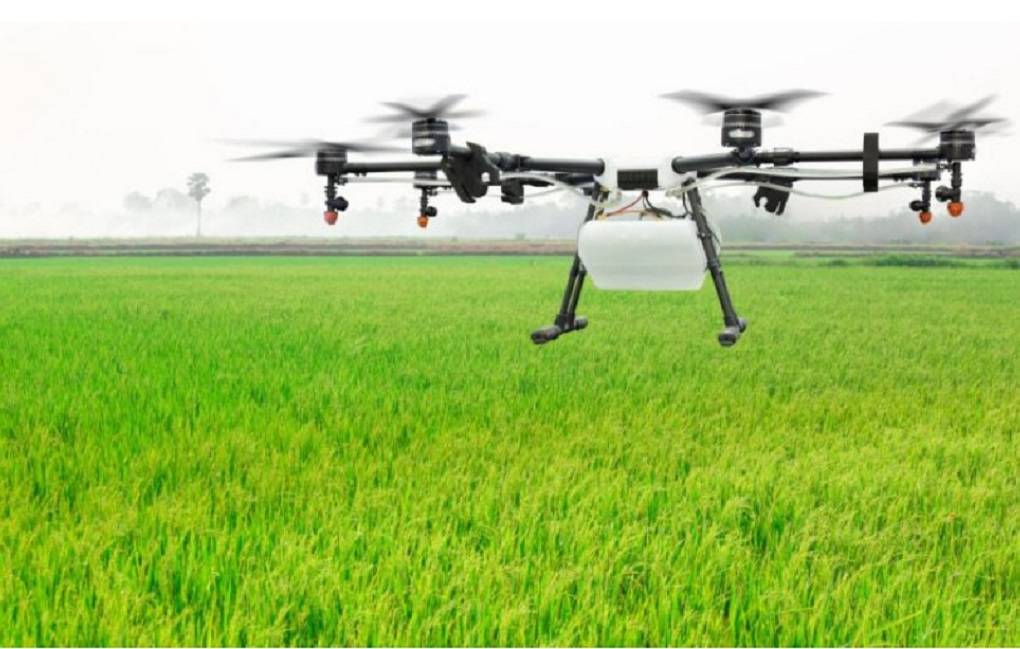
Geospatial technology is a field that is rapidly evolving. Geographic information systems (GIS), global positioning systems (GPS), and remote sensing (RS), all new technologies that aid the user in the collecting, analysis, and interpretation of spatial data, are referred to as geospatial technology (GST). It is concerned with the relationship and state of artificial and natural objects in space, whether on Earth or elsewhere.
In India, geospatial technology has made inroads into a variety of areas, both public and private. Agriculture, telecommunications, oil and gas, environmental management, forestry, public safety, infrastructure, and logistics are among the major sectors in India that use geospatial technology. The geospatial industry is expected to grow by leaps and bounds in the next years as stakeholders from all sectors see the value and long-term cost effectiveness of adopting geospatial tools and technologies.
By 2025, India's GDP is predicted to reach $9-10 trillion, and the country's economy would have transformed into one that is highly industrialised and technologically advanced. To prepare for the anticipated economic expansion, India would need an effective and modern information and knowledge regime. In the future, geospatial technologies will be crucial to information management in India, and uses of this technology can have significant social and national implications. The market for GIS-based technology in India has been growing at such a rapid rate that it is expected to reach USD 10 billion by 2019. (Indian Geospatial Market Report 2009). Several Indian states, including Andhra Pradesh, Karnataka, Rajasthan, and Tamil Nadu, have begun to use geospatial technology to improve governance and management.
Geospatial Technology's Impact on Agriculture
In the Asia-Pacific area, the use of new and modern information, geospatial, and communication technologies (ICTs) for rural and agricultural development has progressed at a rapid pace during the previous decade. Plantation crops are largely surveyed and mapped using geospatial technology. In nations like Malaysia and Indonesia, rice mapping is a big endeavor. Australia is a prominent consumer of geospatial technologies, with remote sensing and geographic information systems (GIS) being particularly popular for mapping crops like sugarcane and oil palms. At the micro-level, geospatial techniques are primarily utilised for mapping groundwater resources, drainage patterns, variable rate fertiliser delivery, and pesticide and insecticide management. Increased yields, resource management, prediction of outcomes, and improved farm practises are all benefits of geospatial technologies in agriculture.
Role in Carbon Sequestration
The act of transferring and securely storing atmospheric CO2 into other long-lived carbon reservoirs that would otherwise be vented or remain in the atmosphere is known as carbon sequestration (CS). The capacity of integrated RS-GIS to do advanced spatial and/or temporal analysis on several layers of high resolution data is its main strength. This aids research activities aimed at controlling the global carbon cycle, mostly by giving useful data and assisting with implementation methods. In CS administration and monitoring, an integrated RS-GIS can be used as a Decision Support System (DSS) tool. The use of an integrated RS-GIS allows for the quantification of climatic and soil conditions across a region's geographical and temporal variability (Niu and Duiker, 2006).
Carbon stocks have been mapped using remote sensing methods such as Synthetic Aperture Radar (SAR), Light Detection and Ranging (LiDaR), and satellite sensors like as Landsat, SPOT, and Ikonos (Goetz et al., 2009). The total soil CS potential in the area, double planted with wheat-corn under a typical tillage regime, was found to range between 0.16-0.43 Pg of C without affecting crop yields significantly (Thomson et al., 2006). The recovery of vegetation increases the CS potential of eroded soils, according to this study. C-Lock, a new system that standardises the estimation of agricultural carbon sequestration credits, was developed using a regional-scale GIS as the operating platform. The association of soil CS potential with soil erosion type, altitude, soil type, and soil parent material was examined using a GIS in a recent experiment to simulate soil CS potential in eroded locations (Shi et al., 2009).
The regeneration of vegetation boosts the CS potential of degraded soils, according to this study. C-Lock, a new system that standardises the estimation of agricultural carbon sequestration credits, was developed using a regional-scale GIS as the operating platform. Century, a biogeochemical model that simulates carbon, nutrient, and water dynamics for various ecosystems, is included in this system. Precision carbon management, an expanding domain of applied science, is made easier with such a technology.
In CS management, the synergistic role of RS and GIS technologies was integrated. Several studies have found that using an integrated RS-GIS method can help with CS management and monitoring strategies. This approach may be used to estimate above- and below-ground biomass, delineate geographic variability, predict potential carbon stocks and revenues, and outline appropriate management strategies for localised and regional scales in climate change mitigation. The use of an integrated RS-GIS strategy for precision carbon management will become increasingly obvious in the near future.
Collection, analysis, and interpretation of geospatial data for agricultural purposes
If the relevant data is not collected and evaluated efficiently, geospatial technology will fail. Several strategies have been developed to achieve this, the majority of them are based on remote sensing. In order to divide a vast farm into management zones, remote sensing is required (Grisso, 2009). Each zone has unique requirements that necessitate the usage of GIS and GPS to meet. As a result, dividing the land into management zones is the first stage in precision farming. The division of this land into zones is mainly based on:
Soil types, pH rates, pest infestation, nutrient availability, soil moisture content, fertility requirements, weather projections, crop traits, and hybrid responses are all used to divide this area into zones.
This information can be found by looking through the records that are available. Most farms have records of soil survey maps, historical crop characteristics, and documents that reflect the region's cropping methods. Aerial and satellite images can also be employed in this procedure. Three characteristics were examined from a Daedalus sensor aboard a NASA aeroplane in the image sample below shot on January 30, 2001. In each image, the individual fields are numbered. The vegetation density is seen in the top image (mainly yellow). The hue contrasts represent crop density, with dark blues and greens indicating lush vegetation and reds indicating bare soil (the "Normalized Difference Vegetation Index," or NDVI). The water distribution was evaluated in the middle figure, with green and blue spots suggesting wet soil and red portions indicating dry soil. The centre image was created using the Daedalus sensor's reflectance and temperature measurements. The bottom image evaluates crop stress, with red and yellow pixels indicating high stress areas. The information gathered from assessing these various parameters enables the farmer to micromanage the administration of water in order to effectively handle varying soil conditions and vegetation growth.
Additionally, current aerial and satellite images of the farm can be generated at various times of the year or seasons. The farmer can use this information to calculate the production of various management zones. At the same time, different zones within the farm's growth and yield trends can be detected.
To improve the efficiency of this procedure, a variety of remote sensing techniques can be applied. Observation with the naked eye is the most popular remote sensing approach that has been used over the years. Any observation made using this method is usually geo-referenced into a GIS database with the help of contemporary technologies. Much of precision agriculture is dependent on image-based remote sensing data, such as detecting the greenness of a field using a technology to determine the productivity/yield of different management zones (Brisco et al, n.d.). The relationship that comes from comparing the reflection of red light and near infrared light is the basis for this technique. Farmers have also benefited from RADARSAT data, which has given them with reliable information on the characteristics that govern soil conditions and crop performance.
The information gathered through remote sensing serves as a source of point data. This dataset may easily be turned into spatial data that depicts the state of all management zones inside the farm using various GIS techniques and tools based on the trends and frequencies that have been recorded. Kriging is an example of a technique for converting remote sensing point data into spatial data (Brisco et al, n.d.). The potential problems that may exist in various management zones can then be determined using spatial data. This provides farmers with the information they need to make informed and effective decisions about how to address current issues and increase overall farm production.
For point data to be relevant to the farmer, it must be kept and analysed once it has been collected. The usage of GIS tools becomes necessary at this point. GIS software may be used to create digital maps that convert geospatial data obtained on the ground into digital representation. Simultaneously, point data collected on the field can now be transformed into spatial data that reflects the entire farm. The collected data is typically presented in either raster or vector formats to effectively differentiate points with different values within the management zones (Brisco et al, n.d.). The raster format creates artificial grids within a map. Various colours are applied to points on the map that have different values. As a result, a user can identify points with similar features and distinguish them from points with different characteristics only by looking at them. This type of data representation is useful in spatial modelling since it demonstrates the relationship between grouped data. The x-axis and y-axis coordinates are used to assign a specific point inside a map in vector format. A boundary is formed by plotting points with comparable features and joining them together. In computerised mapping and spatial database management, this type of data presentation is effective.
After the spatial data has been mapped, it is critical to compare the results with the field notes. This procedure is used to identify any trends or connections that may exist on the ground. An location with a high nutritional content in the soil or one that is heavily infested with parasites may be detected at this point. This variability might take the shape of either uniform or non-uniform variability. With this knowledge, better management approaches may be implemented to boost farming efficiency, ensuring the best use of inputs and maximising output. As a result, the information obtained by remote sensing and GIS can be utilised to make site-specific decisions about fertiliser, herbicides and insecticides, irrigation, and other issues. Most essential, the data collected must be kept in a logical and systematic manner for future reference. This is critical since it will improve the efficacy and efficiency of future surveys.
The primary goal of gathering this information is for a farmer to have a clear grasp of the demands of various parts of the farm in order to maximise production. As this demand grows, the use of automated farm machinery will become more common (Sohne et al, 1994). These devices are required to carry out their tasks perfectly in accordance with the data provided to them. Using GIS and GPS, automated agricultural machinery is now more accurate, safe, and efficient, requiring less human labour to operate and, most significantly, increasing farm yield.
Conclusion
Farmers can better understand their farms' site-specific demands by using remote sensing, GPS, and GIS. They can utilise this information to develop and implement management approaches that assure the best possible utilisation of inputs in order to maximise production and revenues. As a result, geospatial technologies give a farmer with a repository of information that he or she may utilise to make informed decisions that ensure effective and efficient farm management and enhance productivity. To get the most out of their farms, farmers should understand and utilise these technologies in conjunction with their knowledge and expertise.
References
Brisco, B., Brown, R., Hirose, J., McNairn, H. and Staenz, K. (n.d.). Precision Agriculture and the Role of Remote Sensing: A Review. Retrieved on 1st October 2012 from ftp://ftp.geogratis.gc.ca/part6/ess_pubs/219/219370/3520.pdf
Esri (2008). GIS for Sustainable Agriculture. GIS Best Practices. New York: ESRI Publications
Grisso, B. (2009). Precision Farming: A Comprehensive Approach. Retrieved on 1st October 2012 from http://pubs.ext.vt.edu/442/442-500/442-500.html
Sohne, W., Heinze, O. and Groten, E. (1994). Integrated INS/GPS System for High Precision Navigation Applications. Record-IEEE PLANS, Position Location and Navigation Symposium, 35(2): 310-313.
Xiangjian, M. and Gang, L. (2007). Integrating GIS and GPS to Realise Autonomous Navigation of Farm Machinery. New Zealand Journal of Research, 50(1), 807-812
Author Details
-
Smriti Rao1, Akshita Barthwal2 and Raghu Nandan Singh Khatana3
Assistant Professor1 Research Scholar23
Department of Soil Science Integral University Lucknow and department of Soil science and agricultural Chemistry, NAI, SHUATS Prayagraj
Share your comments